Something in-between, a thought that initiated this question, “What is the smallest number between two numbers?”
In the real world, this is an attempt to reveal what’s in the space between two or more visible objects. Numbers form the framework in which the world is built, and the universe seems to follow some set of mathematical rules.
To keep this theory simply as much as possible, there is no ‘empty’ space between two objects at different positions just as it is with numbers – empty space isn’t empty.
If we understand how numbers are built, then we would have a full grasp of the knowledge on how things work around us. For example, the distance between where you’re standing from the wall (or whatever is) before you is not an empty space. There is a form of connection or interaction, not visible to you.
When moving from, let’s say number 0 to 1, you’d notice 1 is not independent of 0, and the numbering system permits the presence of other numbers (in decimal format) to exist in-between. The smallest number between 0 and 1 can not be determined.
It appears that the list of decimals between 0 and 1 is doomed to be incomplete, presumably because the infinity of decimals is greater than that of counting numbers.
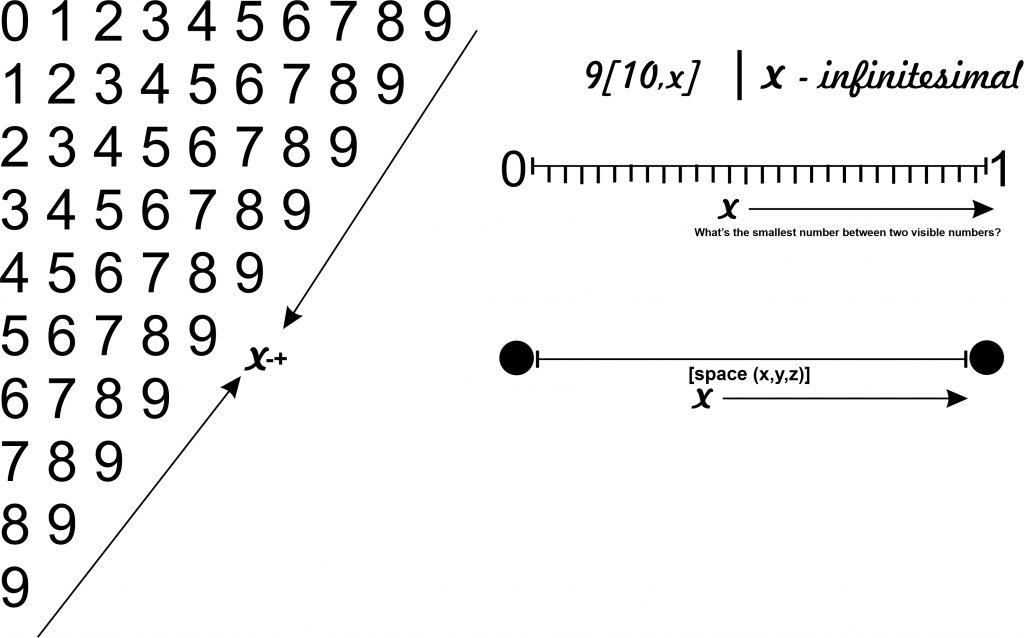
In 1935, Albert Einstein co-authored a paper with his two postdoctoral research associates at the Institute for Advanced Study, Boris Podolsky, and Nathan Rosen. The paper was entitled “Can Quantum-Mechanical Description of Physical Reality Be Considered Complete?” (Einstein et al. 1935) — commonly called EPR.
It presented a striking case where two quantum systems interact in such a way as to link both their spatial coordinates in a certain direction and also their linear momenta (in the same direction), even when the systems are widely separated in space. As a result of this “entanglement”, determining either position or momentum for one system would fix (respectively) the position or the momentum of the other.
According to complementarity when we observe the position of an object, we affect its momentum uncontrollably. Thus we cannot determine both position and momentum precisely.
This idea is based on a statistical prediction of interaction between particles (or waves) in a quantum domain.
Einstein had two reservations about the quantum theory. Firstly, he felt the theory had abdicated the historical task of natural science to provide knowledge of significant aspects of nature that are independent of observers or their observations. Instead, the fundamental understanding of the quantum wave function was that it only treated the outcomes of measurements (via probabilities given by the Born Rule). The theory was simply silent about what, if anything, was likely to be true in the absence of observation.
Secondly, quantum theory was essentially statistical. The probabilities built into the wave function were fundamental and, unlike the situation in classical statistical mechanics, they were not understood as arising from ignorance of fine details. In this sense the theory was indeterministic. Thus Einstein began to probe how strongly the quantum theory was tied to irrealism and indeterminism.
A fundamental fact of quantum mechanics is that we can never know exactly the position and velocity of a particle. Quantum mechanics’ equations for the motion of elementary particles are equations for a wave. The wave only predicts the chance (probability) of finding a particle here or there. It is most likely located where the wave is the highest.
The mechanical universe cannot happen because nature prevents us from accurately knowing both the position and velocity of an elementary particle. Chance did not feel right to many scientists, including Einstein. Some thought there must be a better theory that would remove the uncertainty.
They conclude that quantum mechanics is incomplete. For a complete theory, “every element of the physical reality must have a counterpart in the physical theory.” — The picture is that quantum systems have real states that assign values to certain quantities. Secondly, if quantum mechanics were complete, then incompatible quantities could have simultaneous real values.
The need for a theory that connects and explains everything together is needed. This is where the String theory came in — a theory of everything; from the smallest things that can exist (elementary particles of an atom) to the huge universe.
To understand the string theory, you need to first understand the ideas that formed it. First, Newton’s law of Gravitation — says that there is an attractive force between any two masses. He developed an equation and the mathematics called Calculus to demonstrate it. It was a remarkable idea to explain the force of gravity (field) between two masses that are distant apart (not in contact). The movement of one of the masses can influence the position of the other even though they are not physically connected to each other.
F = GmM / R2
The equation tells us gravity gets stronger if the masses are heavier. The closer the masses are, the stronger the attraction. If R = 0, the force is infinite. That can only happen if the masses have zero radiuses — this points to elementary particles (protons and neutrons). Is the gravitational force between elementary particles infinite? Only string theory solves this problem.
This knowledge is very useful because understanding the behaviour of the smallest building blocks of the Universe underpins our understanding of everything else.
Next, Einstein’s equations which mixed up space with time and energy with mass helped to solve what Classical Physics considered to be separate. In special relativity, space and time mix when speeds approach the speed of light. In general relativity, matter warps space. All of the matter in the universe shapes the universe itself.
The issue is, quantum mechanics was not compatible with relativity. General relativity showed that gravity was due to the warping of space by anything that has mass. Quantum mechanics presented constantly bubbling energy and virtual particles in a vacuum (ignoring gravity). The virtual particles shred up spacetime so badly that the equations of relativity give crazy results. They just do not apply. The variations may be due to virtual particles popping up in the middle of the reaction. Virtual elementary particles are continuously appearing and disappearing everywhere in the universe.
Each particle has an anti-particle. Anti-particles make up anti-matter. If a particle meets its anti-particle, they both annihilate with a burst of pure energy. All of their mass converts to energy. The equations in physics are the same for matter and antimatter. That makes most physicists believe there should be as much antimatter as matter in the universe. Unfortunately, a collision between matter and anti-mater is yet to be seen in our universe. Why is this not happening if the laws of physics are the same for matter and antimatter? Neither quantum mechanics nor string theory has a widely accepted answer.
Quantum mechanics seems so strange. It is concerned with objects far smaller than anything we can see or feel. The number of elementary particles went from just the proton, neutron, and electron to about 200 more — discovered by High-energy accelerators. They get protons or electrons moving near the speed of light and crash them into a target. Exerting that much energy into a tiny spot can create particles never before seen.
Newton’s laws were wonderful. If you knew where everything is and the velocity of everything, then you could accurately calculate the past and the future. Quantum measurements, however, are fuzzy. It is impossible to measure anything without changing other things. The more accurately you measure one, the less accurate the other becomes. This is the uncertainty principle.
Quantum effects happen in our normal-sized world but they are so small that we cannot detect them with the best instrumentation, and we certainly cannot see them.
Quantum mechanics and relativity deal with opposite ends of the universe, sub-atomic particles, and the whole universe. String theory is required to agree with both quantum mechanics and relativity results. Both are correct in where they apply and they are very accurate.
String Theory
String theory is going to seem strange as we do not have any known experience with these realms — the smallest and biggest things in our universe. String theory should agree with the accurate results of quantum mechanics and relativity. String theory is a theory of quantum gravity.
Twelve elementary particles were discovered, eight of those twelve do not occur naturally and appear to be unnecessary, and we focus on four elementary particles that make up our universe which is reduced to one thing — string.
One kind of string vibrating in different modes can generate the elementary particles. Other types of string vibration create the particles that carry force. String theory explains all the forces and particles with one constant, the stiffness of the string.
String theory inherits the quantum mechanics view that forces are due to the exchange of virtual force particles. The four fundamental forces electromagnetic force, strong force, gravity, and weak force hold the world together by the exchange of virtual force-carrying strings. These are respectively, the photon, gluon, graviton, and the W and Z bosons. These strings are vibrating at different notes or frequencies and can have different spin and mass.
The four forces differ in strength and the distance over which they act. They are so different that it is hard to imagine they could arise from different modes of vibration of string. The forces we know personally are electromagnetic and gravity. They both have a long range, which is why we know them; we can feel them. The electromagnetic force is fascinating because it accounts for things as different as lightning, magnets, light, radio, and x-rays. Strong and weak forces are nuclear forces that do not reach outside the nucleus. We do not feel them.
There is a huge difference in strength between gravity and electromagnetic force. The electromagnetic force is 10^+36, a million quadrillion times stronger than gravity. However, that does not seem right — gravity holds us on the Earth but that’s exactly how it is.
Complications of String Theory
The first complication is the change from points in quantum mechanics to string. A point has no size. It has no direction. From all directions, it looks the same, completely spatially symmetric. Strings break that symmetry. A string has length. The length can point in a particular direction. The effect of a force still depends on the direction the force is pointing, but now may also depend on the direction the string is pointing.
When a point is still, it is still. Even if a string stops, it still vibrates near the speed of light. Therefore, strings must always obey the rules of relativity. Strings, like points, also have to obey all normal laws of physics, like conservation of energy, momentum, and angular momentum (spin). When a string moves in spacetime, its spin, mass, charges, and length stay the same.
A string can change shape. When bumping into other strings, strings can split or join the other strings. All this is much more complicated than what points can do. These complications result in many rules that strings must obey. One of the rules is that string cannot move.
Physicists were desperate to solve the problem of making strings move and have string theory work. The only way to make string move was if space had ten dimensions.
How can space have ten dimensions when all we experience are three? Only three of the dimensions are large. The other seven are tiny.
String theory’s extra dimensions all curl up on each other into tiny, complicated, seven-dimensional shapes that look like knots. The knots are about the size of a string. That is so small that no equipment will ever see them. A knot of curled up dimensions exists at every point in space.
We do not notice the knots and bumpiness of space for the same reason we do not notice quantum effects. They are far too small. Take the period at the end of this sentence. The knots in space are much smaller than that.
Until the attempts of String theory to unify all theories (a search for the theory of everything) is understood, we may not have a clear picture of the structure of our universe, and what exists in-between.
However, as much as a valid theory is needed to explain or uncover what’s in-between, the presence of an infinitesimal which connects everything like a web remains undeniable. A little change in any tiny particle has an effect on the whole structure of our universe. We are all connected!
Also published here
# something in-between # something in-between # something in-between # something in-between # something in-between # something in-between # something in-between